Solid-phase peptide synthesis: from standard procedures to the synthesis of difficult sequences
This protocol for solid-phase peptide synthesis (SPPS) is based on the widely used Fmoc/tBu strategy, activation of the carboxyl groups by aminium-derived coupling reagents and use of PEG-modified polystyrene resins. A standard protocol is described, which was successfully applied in our lab for the synthesis of the corticotropin-releasing factor (CRF), >400 CRF analogs and a countless number of other peptides. The 41-mer peptide CRF is obtained within ∼ 80 working hours. To achieve the so-called difficult sequences, special techniques have to be applied in order to reduce aggregation of the growing peptide chain, which is the main cause of failure for peptide chemosynthesis. Exemplary application of depsipeptide and pseudoproline units is shown for synthesizing an extremely difficult sequence, the Asn(15) analog of the WW domain FBP28, which is impossible to obtain using the standard protocol.
This is a preview of subscription content, access via your institution
Access options
Subscribe to this journal
Receive 12 print issues and online access
265,23 € per year
only 22,10 € per issue
Buy this article
- Purchase on SpringerLink
- Instant access to full article PDF
Prices may be subject to local taxes which are calculated during checkout
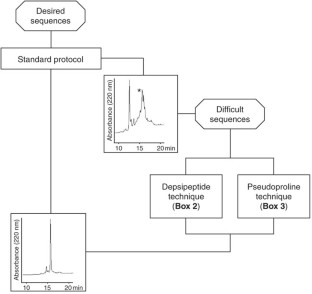
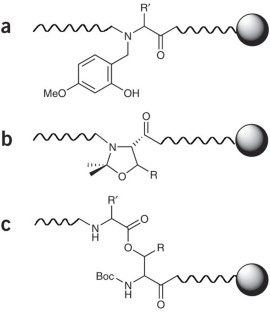
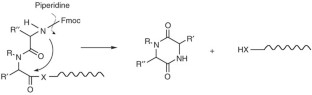
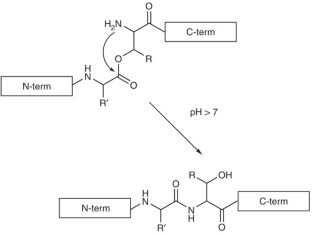
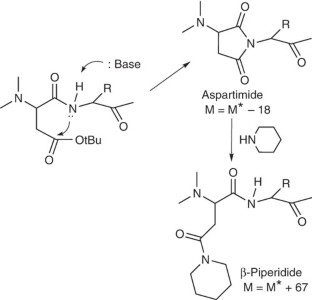
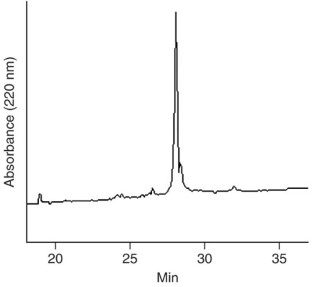
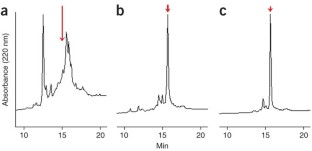
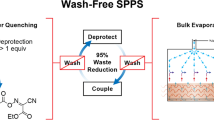
Total wash elimination for solid phase peptide synthesis
Article Open access 09 December 2023
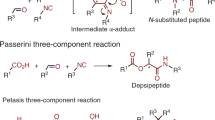
On-resin multicomponent protocols for biopolymer assembly and derivatization
Article 20 January 2021

Rapid automated iterative small-molecule synthesis
Article 29 May 2024
References
- Fischer, E. & Otto, E. Synthesis of the derivatives of some dipeptides. Ber. Deutsch. Chem. Ges.36, 2106–2116 (1903). ArticleCASGoogle Scholar
- Bergmann, M. & Zervas, L. A general procedure of the peptide synthesis. Ber. Deutsch. Chem. Ges.65, 1192–1201 (1932). ArticleGoogle Scholar
- Anderson, G.W., Blodinger, J. & Welcher, A.D. Tetraethylpyrophosphite as a reagent for peptide syntheses. J. Am. Chem. Soc.74, 5309–5311 (1952). ArticleCASGoogle Scholar
- Siffert, R.H. & du Vigneaud, V. A new synthesis of carnosine, with some observations on the splitting of the benzyl group from carbobenzoxy derivatives and from benzylthioethers. J. Biol. Chem.108, 753–761 (1935). Google Scholar
- Merrifield, R.B. History of protein synthesis. In Houben-Weyl. Methods of Organic Chemistry. Vol. E 22b: Synthesis of Peptides and Peptidomimetics (eds. Goodman, M., Felix, A., Moroder, L. & Toniolo, C.) 3–41 (Thieme, Stuttgart, New York, 2002). Google Scholar
- Du Vigneaud, V., Ressler, C., Swan, J.M., Roberts, C.W. & Katsoyannis, P.G. The synthesis of oxytocin. J. Am. Chem. Soc.76, 3115–3121 (1954). ArticleCASGoogle Scholar
- Merrifield, R.B. Solid phase peptide synthesis. 1. Synthesis of a tetrapeptide. J. Am. Chem. Soc.85, 2149–2154 (1963). ArticleCASGoogle Scholar
- Atherton, E., Clive, D.L. & Sheppard, R.C. Polyamide supports for polypeptide-synthesis. J. Am. Chem. Soc.97, 6584–6585 (1975). ArticleCASGoogle Scholar
- Atherton, E., Brown, E. & Sheppard, R.J. Internal association in solid-phase peptide-synthesis—synthesis of cytochrome-C residues 66-104 on polyamide supports. J. Chem. Soc. Chem. Commun. 1151–1152 (1981).
- Bayer, E. Towards the chemical synthesis of proteins. Angew. Chem. Int. Ed. Engl.30, 113–129 (1991). ArticleGoogle Scholar
- Zalipsky, S., Chang, J.L., Albericio, F. & Barany, G. Preparation and applications of polyethylene glycol-polystyrene graft resin supports for solid-phase peptide-synthesis. React. Polym.22, 243–258 (1994). ArticleCASGoogle Scholar
- Albericio,, F. & Giralt, E. Handles and supports. In Houben-Weyl. Methods of Organic Chemistry. Vol. E 22a: Synthesis of Peptides and Peptidomimetics (eds. Goodman, M., Felix, A., Moroder, L. & Toniolo, C.) 685–709 (Thieme, Stuttgart, New York, 2002). Google Scholar
- Carpino, L.A. Oxidative reactions of hydrazines. 2. Isophthalimides. New protective groups on nitrogen. J. Am. Chem. Soc.79, 98–101 (1957). ArticleCASGoogle Scholar
- Stewart, J.M. Protection strategies. In Houben-Weyl. Methods of Organic Chemistry. Vol. E 22a: Synthesis of Peptides and Peptidomimetics (eds. Goodman, M., Felix, A., Moroder, L. & Toniolo, C.) 726–739 (Thieme, Stuttgart, New York, 2002. Google Scholar
- Carpino, L.A. 9-Fluorenylmethoxycarbonyl function, a new base-sensitive amino-protecting group. J. Am. Chem. Soc.92, 5748 (1970). ArticleCASGoogle Scholar
- Chang, C.D. & Meienhofer, J. Solid-phase peptide-synthesis using mild base cleavage of N-alphafluorenylmethyloxycarbonylamino acids, exemplified by a synthesis of dihydrosomatostatin. Int. J. Pept. Protein Res.11, 246–249 (1978). ArticleCASGoogle Scholar
- Atherton, E. & Wellings, D.A. 9-Fluorenylmethoxycarbonyl/tert-butyl strategy. In Houben-Weyl. Methods of Organic Chemistry. Vol. E 22a: Synthesis of Peptides and Peptidomimetics (eds. Goodman, M., Felix, A., Moroder, L. & Toniolo, C.) 740–754 (Thieme, Stuttgart, New York, 2002). Google Scholar
- Sheehan, J.C. & Hess, G.P. A new method of forming peptide bonds. J. Am. Chem. Soc.77, 1067–1068 (1955). ArticleCASGoogle Scholar
- Wieland, T., Kern, W. & Sehring, R. Über anhydride von acylierten aminosäuren. Justus Liebigs Ann. Chem.569, 117–121 (1950). ArticleCASGoogle Scholar
- Schwyzer, R., Iselin, B. & Feurer, M. Über aktivierte ester. 1. Aktivierte ester der hippursäure und ihre umsetzungen mit benzylamin. Helv. Chim. Acta38, 69–79 (1955). ArticleCASGoogle Scholar
- Coste, J. Phosphonium salts. In Houben-Weyl. Methods of Organic Chemistry. Vol. E 22a: Synthesis of Peptides and Peptidomimetics (eds. Goodman, M., Felix, A., Moroder, L. & Toniolo, C.) 538–554 (Thieme, Stuttgart, New York, 2002). Google Scholar
- Bienert, M., Henklein, P., Beyermann, M. & Carpino, L. A. Uronium/guanidinium salts. In Houben-Weyl. Methods of Organic Chemistry. Vol. E 22a: Synthesis of Peptides and Peptidomimetics (eds. Goodman, M., Felix, A., Moroder, L. & Toniolo, C.) 555–580 (Thieme, Stuttgart, New York, 2002). Google Scholar
- Atherton, E. & Sheppard, R.C. Solid Phase Peptide Synthesis: A Practical Approach (IRL Press, Oxford, UK, 1999). Google Scholar
- Pennington, M.W. & Dunn, B.M. Peptide Synthesis Protocols (Humana Press, Totowa, New Jersey, 1994). BookGoogle Scholar
- Fields, G.B. Solid-Phase Peptide Synthesis (Academic Press, New York, 1997). Google Scholar
- Lloyd-Williams, P., Albericio, F. & Giralt, E. Chemical Approaches to the Synthesis of Peptides and Proteins (CRC Press, Boca Raton, Florida, 1997). Google Scholar
- Chan, W.C. & White, P.D. Fmoc Solid Phase Peptide Synthesis: A Practical Approach (Oxford University Press, Oxford, UK, 2000). Google Scholar
- Sewald, N. & Jakubke,, H.-D. Peptides: Chemistry and Biology (Wiley-VCH, Weinheim, 2002). BookGoogle Scholar
- Goodman, M., Felix, A., Moroder, L. & Toniolo, C. (eds.) Houben-Weyl. Methods of Organic Chemistry. Vol. E 22a–e: Synthesis of Peptides and Peptidomimetics (Thieme, Stuttgart, New York, 2002). Google Scholar
- Amblard, M., Fehrentz, J.A., Martinez, J. & Subra, G. Methods and protocols of modern solid phase peptide synthesis. Mol. Biotechnol.33, 239–254 (2006). ArticleCASGoogle Scholar
- Dawson, P.E. & Kent, S.B. Synthesis of native proteins by chemical ligation. Annu. Rev. Biochem.69, 923–960 (2000). ArticleCASGoogle Scholar
- Bray, B.L. Large-scale manufacture of peptide therapeutics by chemical synthesis. Nat. Rev. Drug Discovery2, 587–593 (2003). ArticleCASGoogle Scholar
- Vale, W., Spiess, J., Rivier, C. & Rivier, J. Characterization of a 41-residue ovine hypothalamic peptide that stimulates secretion of corticotropin and beta-endorphin. Science213, 1394–1397 (1981). ArticleCASGoogle Scholar
- Dauzenberg, F.M. & Hauger, R.L. The CRF peptide family and their receptors: yet more partners discovered. Trends Pharmacol. Sci.23, 71–77 (2002). ArticleGoogle Scholar
- Beyermann, M., Fechner, K., Furkert, J., Krause, E. & Bienert, M. A single-point slight alteration set as a tool for structure-activity relationship studies of ovine corticotropin- releasing factor. J. Med. Chem.39, 3324–3330 (1996). ArticleCASGoogle Scholar
- Beyermann, M. et al. A role for a helical connector between two receptor binding sites of a long-chain peptide hormone. J. Biol. Chem.275, 5702–5709 (2000). ArticleCASGoogle Scholar
- Beyermann, M. et al. Achieving signalling selectivity of ligands for the corticotropin-releasing factor type 1 receptor by modifying the agonist's signalling domain. Br. J. Pharmacol.151, 851–859 (2007). ArticleCASGoogle Scholar
- Rivier, J.E. & Miranda, M.T.M. Solid-phase peptide synthesis at elevated temperature. In Houben-Weyl. Methods of Organic Chemistry. Vol. E 22a: Synthesis of Peptides and Peptidomimetics (eds. Goodman, M., Felix, A., Moroder, L. & Toniolo, C.) 806–813 (Thieme, Stuttgart, New York, 2002). Google Scholar
- Kaiser, E., Colescot, R.L., Bossinge, C.D. & Cook, P.I. Color test for detection of free terminal amino groups in solid-phase synthesis of peptides. Anal. Biochem.34, 595–598 (1970). ArticleCASGoogle Scholar
- Carpino, L.A., Beyermann, M., Wenschuh, H. & Bienert, M. Peptide synthesis via amino acid halides. Acc. Chem. Res.29, 268–274 (1996). ArticleCASGoogle Scholar
- Gilon, C., Dechantsreiter, M.A., Burkhart, F., Friedler, A. & Kessler, H. Synthesis of N-alkylated peptides. In Houben-Weyl. Methods of Organic Chemistry. Vol. E 22c: Synthesis of Peptides and Peptidomimetics (eds. Goodman, M., Felix, A., Moroder, L. & Toniolo, C.) 215–271 (Thieme, Stuttgart, New York, 2002). Google Scholar
- Hyde, C., Johnson, T., Owen, D., Quibell, M. & Sheppard, R.C. Some “difficult sequences” made easy. A study of interchain association in solid-phase peptide synthesis. Int. J. Peptide Protein Res.43, 431–440 (1994). ArticleCASGoogle Scholar
- Fields, C. & Fields, G.B. Solvents for solid-phase peptide synthesis. In Peptide Synthesis Protocols (eds. Penningten, M.W. & Dunn, B.M.) 29–40 (Humana Press, Totowa, New Jersey, 1994). ChapterGoogle Scholar
- Beyermann, M. & Bienert, M. Synthesis of difficult peptide sequences: a comparison of Fmoc- and Boc-technique. Tetrahedron Lett.33, 3745–3748 (1992). ArticleCASGoogle Scholar
- Narita, M., Fukunaga, T., Wakabayashi, A., Ishikawa, K. & Nakano, H. Syntheses and properties of tertiary peptide bond-containing polypeptides. 1. Syntheses and properties of oligo( L -leucine)S containing proline of glycyl-N-(2,4-dimethoxybenzyl)- L -leucine residues. Int. J. Peptide Protein Res.23, 306–314 (1984). ArticleCASGoogle Scholar
- Johnson, T., Quibell, M. & Sheppard, R.C. N,O-bis Fmoc derivatives of N-(2-hydroxy-4-methoxybenzyl)-amino acids: useful intermediates in peptide synthesis. J. Pept. Sci.1, 11–25 (1995). ArticleCASGoogle Scholar
- Quibell, M., Turnell, W.G. & Johnson, T. Preparation and purification of beta-amyloid (1-43) via soluble, amide backbone protected intermediates. J. Org. Chem.59, 1745–1750 (1994). ArticleCASGoogle Scholar
- Wöhr, T. & Mutter, M. Pseudo-prolines in peptide synthesis: direct insertion of serine and threonine-derived oxazolidines in dipeptides. Tetrahedron Lett.36, 3847–3848 (1995). ArticleGoogle Scholar
- Wöhr, T. et al. Pseudo-prolines as a solubilizing, structure-disrupting protection technique in peptide synthesis. J. Am. Chem. Soc.118, 9218–9227 (1996). ArticleGoogle Scholar
- Toniolo, C., Bonora, G.M., Mutter, M. & Pillai, V.N.R. Linear oligopeptides. 78. The effect of the insertion of a proline residue on the solution conformation of host peptides. Macromol. Chem. Phys.182, 2007–2014 (1981). ArticleCASGoogle Scholar
- Carpino, L.A. et al. Synthesis of “difficult” peptide sequences: application of a depsipeptide technique to the Jung-Redemann 10- and 26-mers and the amyloid peptide Aβ(1-42). Tetrahedron Lett.45, 7519–7523 (2004). ArticleCASGoogle Scholar
- Mutter, M. et al. Switch peptides in statu nascendi: induction of conformational transitions relevant to degenerative diseases. Angew. Chem. Int. Ed. Engl.43, 4172–4178 (2004). ArticleCASGoogle Scholar
- Sohma, Y., Sasaki, M., Hayashi, Y., Kimura, T. & Kiso, Y. Design and synthesis of a novel water-soluble Aβ(1-42) isopeptide: an efficient strategy for the preparation of Alzheimer's disease-related peptide, Aβ(1-42), via O-N intramolecular acyl migration reaction. Tetrahedron Lett.45, 5965–5968 (2004). ArticleCASGoogle Scholar
- Coin, I. et al. Depsipeptide methodology for solid-phase peptide synthesis: circumventing side reactions and development of an automated technique via depsidipeptide units. J. Org. Chem.71, 6171–6177 (2006). ArticleCASGoogle Scholar
- Sohma, Y. et al. 'O-Acyl isopeptide method' for the efficient synthesis of difficult sequence-containing peptides: use of 'O-acyl isodipeptide unit'. Tetrahedron Lett.47, 3013–3017 (2006). ArticleCASGoogle Scholar
- Coin, I., Schmieder, P., Bienert, M. & Beyermann, M. The depsipeptide technique applied to peptide segment condensation: scope and limitations. J. Pept. Sci. DOI: 10.1002/psc.928 (2007).
- Taniguchi, A. et al. 'O-Acyl isopeptide method' for peptide synthesis: solvent effects in the synthesis of Aβ1-42 isopeptide using 'O-acyl isodipeptide unit'. J. Pept. Sci. DOI: 10.1002/psc.905 (2007).
- Pedroso, E., Grandas, A., de las Heras, X., Eritja, R. & Girald, E. Diketopiperazine formation in solid-phase peptide-synthesis using P-alkoxybenzyl ester resins and Fmoc-amino acids. Tetrahedron Lett.27, 743–746 (1986). ArticleCASGoogle Scholar
- Carpino, L.A. et al. New family of base- and nucleophile-sensitive amino-protecting groups. A Michael-acceptor-based deblocking process. Practical utilization of the 1,1-dioxobenzo[b]thiophene-2-ylmethyloxycarbonyl (Bsmoc)group. J. Am. Chem. Soc.119, 9915–9916 (1997). ArticleCASGoogle Scholar
- Fujino, M., Wakimasu, M., Shinagawa, S., Kitada, C. & Yajima, H. Synthesis of the nonacosapeptide corresponding to mammalian glucagons. Chem. Pharm. Bull.26, 539–548 (1978). ArticleCASGoogle Scholar
- Marcias, M.J., Gervais, V., Civera, C. & Oschkinat, H. Structural analysis of WW domains and design of a WW prototype. Nat. Struct. Biol.7, 375–379 (2000). ArticleGoogle Scholar
- Tremmel, S. et al. C-13-labeled tyrosine residues as local IR probes for monitoring conformational changes in peptides and proteins. Angew. Chem. Int. Ed. Engl.44, 4631–4635 (2005). ArticleCASGoogle Scholar
- Nicolás, E., Pedroso, E. & Girald, E. Formation of aspartimide peptides in Asp-Gly sequences. Tetrahedron Lett.30, 497–500 (1989). ArticleGoogle Scholar
- Dölling, R. et al. Piperidine-mediated side product formation for Asp(OtBu)-containing peptides. J. Chem. Soc. Chem. Commun. 853–854 (1994).
- Offer, J., Quibell, M. & Johnson, T. On-resin solid-phase synthesis of asparagine N-linked glycopeptides: use of N-(2-acetoxy-4-methoxybenzyl)(AcHmb) aspartyl amide-bond protection to prevent unwanted aspartimide formation. J. Chem. Soc. Perkin Trans.1, 175–182 (1996). ArticleGoogle Scholar
- Wade, J.D., Bedford, J., Sheppard, R.C. & Tregear, G.W. DBU as an N-alpha-deprotecting reagent for the fluorenylmethoxycarbonyl group in continuous flow solid-phase peptide synthesis. Pept. Res.4, 194–199 (1991). CASGoogle Scholar
- Kates, S.A., Solé, N.A., Beyermann, M., Barany, G. & Albericio, F. Optimized preparation of deca( L -alanyl)- L -valinamide by 9-fluorenylmethyloxycarbonyl (Fmoc) solid-phase synthesis on polyethylene glycol-polystyrene (PEG-PS) graft supports, with 1,8-diazobicyclo[5.4.0]-undec-7-ene (DBU) deprotection. Pept. Res.9, 106–113 (1996). CASPubMedGoogle Scholar
- Thaler, A., Seebach, D. & Cardinaux, F. Improvement of degree of resin swelling and of efficiency of coupling in solid-phase synthesis. Helv. Chim. Acta74, 628–643 (1991). ArticleCASGoogle Scholar
- Pugh, K.C., York, E.J. & Stewart, J.M. Effects of resin swelling and substitution on solid phase synthesis. Int. J. Pept. Protein Res.40, 208–213 (1992). ArticleCASGoogle Scholar
- Pennington, M.W. & Byrnes, M.E. Procedures to improve difficult couplings. In Peptide Synthesis Protocols (eds. Pennington, M.W. & Dunn, B.M.) 1–16 (Humana Press, Totowa, New Jersey, 1994). ChapterGoogle Scholar
- Wade, J.D., Mathieu, M.N., Macris, M. & Tregear, G.W. Base-induced side reactions in Fmoc-solid phase peptide synthesis: minimization by use of piperazine as N-alpha-deprotection reagent. Lett. Pept. Sci.7, 107–112 (2000). CASGoogle Scholar
- Alsina, J., Giralt, E. & Albericio, F. Use of N-tritylamino acids and PyAOP for the supression of diketopiperazine formation in Fmoc/(t)Bu solid-phase peptide synthesis using alkoxybenzyl ester anchoring linkages. Tetrahedron Lett.37, 4195–4198 (1996). ArticleCASGoogle Scholar
- Schnölzer, M., Alewood, P., Jones, A., Alewood, D. & Kent, S.B.H. In situ neutralization in Boc-chemistry solid-phase peptide synthesis—rapid, high-yield assembly of difficult sequences. Int. J. Peptide Protein Res.40, 180–193 (1992). ArticleGoogle Scholar
- Krause, E. et al. Studies on thioether modifications: S-oxidation, S-oxide reduction and regeneration of methionine peptides from their S-benzyl-sulfonium derivatives. In Peptides: Chemistry and Biology (eds. Smith, J.A. & Rivier, J.E.) 478–479 (ESCOM, Leiden, the Netherlands, 1992). ChapterGoogle Scholar
Acknowledgements
We gratefully acknowledge contributions of S. Tremmel, E. Krause, C.D. Sferdean and L.A. Carpino. We thank A. Klose and D. Krause for technical assistance. This work was supported by the Deutsche Forschungsgemeinschaft, grant no. FOR 299/2-2 TP2 and Be 1434/5-2.